2nd Weekly Report from the SONNE expedition SO289
(deutsche Version weiter unten)
Progress:
After a delay of nearly five days, we were able to leave the port of Talcahuano on February 22 and start the cruise SO289. We are now in international waters and will keep sailing west towards New Zealand and New Caledonia. We are twelve days into the cruise, and are sampling at station 17. The weather is very kind to us with very low winds and pleasant temperatures. Since three days, all the Corona restrictions have been lifted on board and we can go about our daily activities without restrictions.
We have established a very efficient routine of equipment deployment and sampling. The team on board the Sonne is very organised and effective. Each day we sample in detail the water column from the surface ocean to the seafloor, and collect waters and particles. We use a titanium CTD rosette frame for contamination prone elements, and also for sampling of microbial communities. The stainless steel CTD frame is used for non-contamination prone sampling of elements and isotopes like radium, thorium, uranium, rare earths and neodymium. At two or three degrees spacing, we occupy a station at which we spend about six hours with the deployments of the titanium and stainless steel CTDs. Every three days, at our “superstations”, we also add an additional stainless steel CTD cast, and deploy up to eleven in situ pumps to a depth of 1100 metres for particle collection. At the “superstations” we spend up to twelve hours. Thorsten Schott, Dennis Köhler and Florian Evers are working hard every day in deploying the CTDs and in situ pumps.
East Pacific Rise:
The South Pacific hosts large underwater volcanoes with hydrothermal vents, which emit hot fluids into the deep ocean at about 2500 metres water depth along the East Pacific Rise (EPR). The East Pacific Rise is a fast spreading ridge system and there are reports of plumes travelling up to 4000 km from the vent sites. The hydrothermal plumes contain high concentrations of iron and other elements that are required by phytoplankton in the surface ocean for their growth. The plumes of iron in the South Pacific deep waters are thought to be transported southwards and to reach the surface waters in the Southern Ocean, which is the largest region in the world’s ocean with phytoplankton growth limitation by iron. In the coming days we will assess the hydrothermal iron inputs and use tracers of helium isotopes and radium to assess hydrothermal plume fluxes and movements. We will employ biogeochemical modelling approaches after the cruise to determine their impact of plume derived iron on the phytoplankton productivity in the Southern Ocean.
We are now making a small detour north towards 31.5°S to have the highest chance of successfully sampling the hydrothermal plume systems of the East Pacific Rise. In case we would have remained on the 32.5°S latitude transect, then we would have crossed the Juan Fernandez microplate, with a lower chance of encountering hydrothermal plumes. We will now occupy a “superstation” in two days time at 31.5°S and 111.9°W, where we will sample in detail the characteristics of the non-buoyant plume system over the East Pacific Rise.
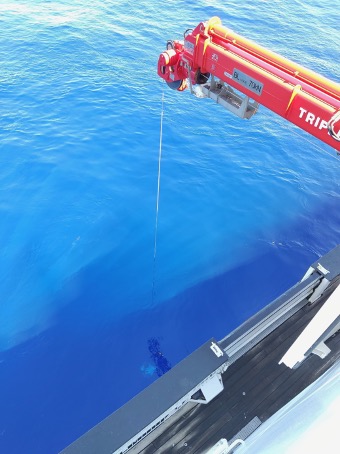
Biological Carbon Pump:
The South Pacific Ocean is one of the most remote and least explored ocean regions of our planet. It also has the largest ocean area of ultra-low productivity, resulting in crystal clear blue waters with very few phytoplankton cells. The low productivity is thought to be caused by a low supply of nutrients like nitrate, and also of trace elements like iron and cobalt, which are all required for phytoplankton growth. The low productivity will likely result in a low uptake of atmospheric CO2 by phytoplankton in this part of the world’s ocean.
We are assessing the transfer of carbon (and trace elements) to the deep ocean, using the 234Th (Thorium) disequilibrium technique. The debris of dead phytoplankton cells sinks from the surface ocean to greater depth. Typically, ocean regions with a high primary productivity will have a higher sinking flux of organic matter. The very low productivity in the South Pacific Ocean is expected to result in a low transfer of particles to the deep ocean, and therefore the uptake of atmospheric CO2 by biological processes is low. The overall process of atmospheric CO2 uptake by phytoplankton, and the transfer of this carbon to depth is called the biological carbon pump. We are assessing the efficiency of the biological carbon pump through the removal in the upper ocean of the 234Th (thorium) isotope by adsorption onto sinking particles; 234Th is produced in the ocean from 238U (uranium) decay.
In our ocean, 238U is homogeneously distributed, and a naturally abundant isotope. Uranium does not interact with particles and is long-lived with a half-life of several million years, decaying to 234Th. 234Th is much less stable with a half-life of only 24.1 days and is highly particle reactive. This means, in contrast to 238U, 234Th readily adsorbs onto surfaces of particles and is removed by the sinking particles to the deep ocean.
We make use of a very simple physical rule: the activity (nuclear decays per minute) of the parent radionuclide (238U) and that of the daughter nuclide (decay product 234Th) is the same if both nuclides are still present within the system observed. In case we observe that the activity of 234Th in the surface ocean is lower than that of 238U, we know that some 234Th has been transported from the surface waters. And that happens exclusively through sinking particles. We therefore determine how much 234Th is transported to depth in association with sinking organic particles, and we can calculate our organic particle flux from the depletion 234Th activity over depths up to 400 m. We use in situ pumps to collect particles for 234Th and particulate carbon analysis, and in addition we sample waters for dissolved 234Th analysis (and also 238U). The sampling and counting of the 234Th isotopes takes place at sea by our student helper Stephan Hamisch, and we will count the samples again once we are back at GEOMAR.
RV Sonne at sea 31.5°S/103.0°W
Eric Achterberg
GEOMAR Helmholtz Centre for Ocean Research Kiel/University of Kiel
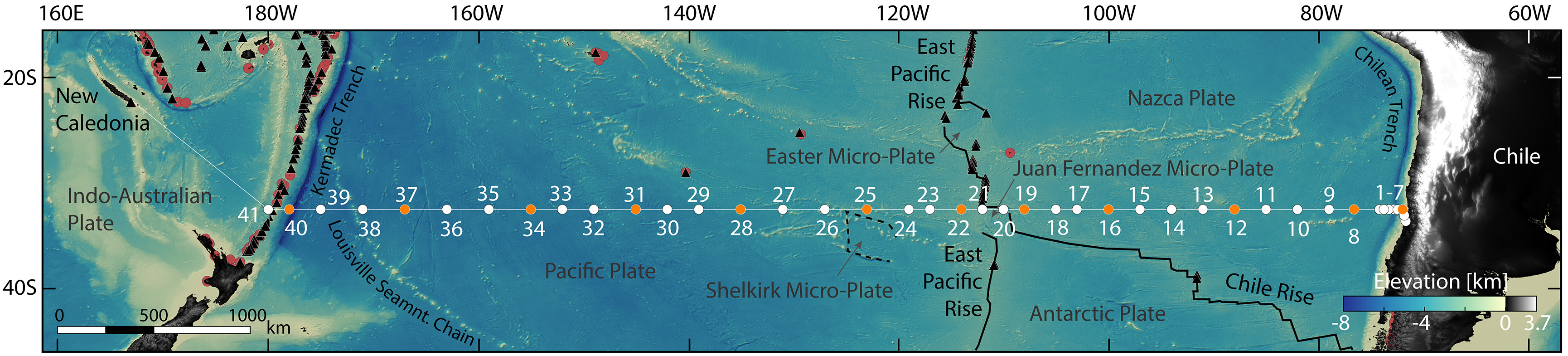
Auf dem Weg zum ostpazifischen Rücken und zur Bewertung der Effizienz der biologischen Kohlenstoffpumpe
Zweiter Wochenbericht der SONNE-Expedition SO289
Fortschritte:
Nach einer Verspätung von fast fünf Tagen konnten wir am 22. Februar den Hafen von Talcahuano verlassen und die Reise SO289 beginnen. Wir befinden uns nun in internationalen Gewässern und werden weiter nach Westen in Richtung Neuseeland und Neukaledonien fahren. Nach zwölf Tagen Fahrt sind wir an der Station 17 angelangt. Das Wetter ist uns sehr wohlgesonnen mit sehr wenig Wind und angenehmen Temperaturen. Seit drei Tagen sind alle Corona-Beschränkungen an Bord aufgehoben, und wir können unseren täglichen Aktivitäten ohne Einschränkungen nachgehen.
Wir haben eine sehr effiziente Routine für den Einsatz der Ausrüstung und die Probenahme eingeführt. Das Team an Bord der Sonne ist sehr gut organisiert und effizient. Jeden Tag beproben wir detailliert die Wassersäule von der Oberfläche bis zum Meeresboden und sammeln Wasser und Partikel. Wir verwenden einen CTD-Rosettenrahmen aus Titan für kontaminationsanfällige Elemente und auch für die Beprobung mikrobieller Gemeinschaften. Der CTD-Rahmen aus Edelstahl wird für nicht-kontaminationsanfällige Probenahmen von Elementen und Isotopen wie Radium, Thorium, Uran, seltene Erden und Neodym verwendet. Im Abstand von zwei oder drei Grad haben eine Station eingerichtet, an der wir etwa sechs Stunden mit den Einsätzen der Titan- und Edelstahl-CTDs verbringen. Alle drei Tage setzen wir an unseren “Superstationen” eine zusätzliche Edelstahl-CTD ein und bringen bis zu elf In-situ-Pumpen bis zu einer Tiefe von 1100 Metern zur Partikelsammlung aus. An den “Superstationen” verbringen wir bis zu zwölf Stunden. Thorsten Schott, Dennis Köhler und Florian Evers arbeiten jeden Tag hart daran, die CTDs und In-situ-Pumpen auszubringen.
Ostpazifischer Rücken:
Der Südpazifik beherbergt große Unterwasservulkane mit hydrothermalen Schloten, die entlang des Ostpazifischen Rückens in etwa 2500 Metern Wassertiefe heiße Flüssigkeiten in die Tiefsee ausstoßen. Der Ostpazifische Rücken ist ein sich schnell ausbreitendes Rückensystem, und es gibt Berichte über Ausströmungen, die bis zu 4000 Kilometer von den Schloten entfernt sind. Die hydrothermalen Ströme enthalten hohe Konzentrationen von Eisen und anderen Elementen, die das Phytoplankton im Oberflächenozean für sein Wachstum benötigt. Man geht davon aus, dass die Eisenfahnen in den Tiefen des Südpazifiks nach Süden transportiert werden und die Oberflächengewässer im Südlichen Ozean erreichen, der größten Region des Weltozeans, in der das Phytoplanktonwachstum durch Eisen begrenzt wird. In den kommenden Tagen werden wir die hydrothermalen Eiseneinträge untersuchen und Tracer von Heliumisotopen und Radium verwenden, um die Flüsse und Bewegungen der hydrothermalen Fahnen zu beurteilen. Nach der Fahrt werden wir biogeochemische Modellierungsansätze anwenden, um die Auswirkungen des Eiseneintrags aus den Plumes auf die Produktivität des Phytoplanktons im Südlichen Ozean zu bestimmen.
Wir machen jetzt einen kleinen Abstecher nach Norden in Richtung 31,5°S, um die besten Chancen zu haben, die hydrothermalen Fahnensysteme des Ostpazifik-Anstiegs erfolgreich zu beproben. Wären wir auf dem 32,5°S Breitengrad-Transekt geblieben, hätten wir die Juan Fernandez Mikroplatte überquert, mit einer geringeren Chance auf hydrothermale Plumes zu stoßen. Wir werden nun in zwei Tagen eine “Superstation” bei 31,5°S und 111,9°W besetzen, wo wir die Eigenschaften des nicht-schwimmenden Plumesystems über dem ostpazifischen Rücken im Detail untersuchen werden.
Biologische Kohlenstoffpumpe:
Der Südpazifik ist eine der abgelegensten und am wenigsten erforschten Meeresregionen unseres Planeten. Hier befindet sich auch das größte Meeresgebiet mit extrem niedriger Produktivität, was zu kristallklarem, blauem Wasser mit sehr wenigen Phytoplanktonzellen führt. Man nimmt an, dass die niedrige Produktivität auf eine geringe Versorgung mit Nährstoffen wie Nitrat und Spurenelementen wie Eisen und Kobalt zurückzuführen ist, die für das Wachstum des Phytoplanktons erforderlich sind. Die geringe Produktivität wird wahrscheinlich zu einer geringen Aufnahme von atmosphärischem CO2 durch das Phytoplankton in diesem Teil des Weltozeans führen.
Wir bewerten den Transfer von Kohlenstoff (und Spurenelementen) in die Tiefsee mit Hilfe der 234Th (Thorium) Ungleichgewichtstechnik. Überreste abgestorbener Phytoplanktonzellen sinken von der Meeresoberfläche in größere Tiefen ab. In der Regel haben Ozeanregionen mit hoher Primärproduktivität einen höheren Fluss an organischen Stoffen, der in die Tiefe sinkt. Die sehr niedrige Produktivität des Südpazifiks dürfte zu einem geringen Transfer von Partikeln in die Tiefsee führen, so dass die Aufnahme von atmosphärischem CO2 durch biologische Prozesse gering ist. Der Gesamtprozess der Aufnahme von atmosphärischem CO2 durch das Phytoplankton und die Weitergabe dieses Kohlenstoffs in die Tiefe wird als biologische Kohlenstoffpumpe bezeichnet. Wir bewerten die Effizienz der biologischen Kohlenstoffpumpe anhand der Entfernung des Isotops 234Th (Thorium) im oberen Ozean durch Adsorption an sinkende Partikel; 234Th entsteht im Ozean aus dem Zerfall von 238U (Uran).
In allen unseren Ozeanen ist 238U gleichmäßig verteilt und ein natürlich reichlich vorhandenes Isotop. Uran geht keine Wechselwirkung mit Partikeln ein und ist mit einer Halbwertszeit von mehreren Millionen Jahren sehr langlebig, wobei es zu 234Th zerfällt. 234Th ist mit einer Halbwertszeit von nur 24,1 Tagen weit weniger stabil und sehr partikelreaktiv. Das bedeutet, dass 234Th im Gegensatz zu 238U leicht an der Oberfläche von Partikeln adsorbiert und von den sinkenden Partikeln in die Tiefsee transportiert wird.
Wir machen uns eine sehr einfache physikalische Regel zunutze: Die Aktivität (Kernzerfälle pro Minute) des Elternradionuklids (238U) und des Tochternuklids (Zerfallsprodukt 234Th) ist gleich, wenn beide Nuklide im beobachteten System noch vorhanden sind. Wenn wir beobachten, dass die Aktivität von 234Th im Oberflächenozean geringer ist als die von 238U, wissen wir, dass etwas 234Th aus dem Oberflächenwasser transportiert wurde. Und das geschieht ausschließlich durch absinkende Partikel. Wir bestimmen daher, wie viel 234Th in Verbindung mit sinkenden organischen Partikeln in die Tiefe transportiert wird, und wir können unseren organischen Partikelfluss aus der Abreicherung der 234Th-Aktivität in Tiefen bis zu 400 Metern berechnen. Wir verwenden In-situ-Pumpen, um Partikel für die Analyse von 234Th und partikulärem Kohlenstoff zu sammeln, und zusätzlich nehmen wir Wasserproben für die Analyse von gelöstem 234Th (und auch 238U). Die Probenahme und Zählung der 234Th-Isotope erfolgt auf See durch unseren studentischen Helfer Stephan Hamisch, und wir werden die Proben erneut zählen, wenn wir wieder am GEOMAR sind.
FS SONNE auf See 31.5°S/103.0°W
Eric Achterberg
GEOMAR Helmholtz-Zentrum für Ozeanforschung Kiel/Universität Kiel