by Elena Schiller, AWI
(deutsch s. u.)
Essentials of deep-sea research technology: The first thing I learnt upon entering a deep sea technology workshop was “non-corrosive steel is non-corroded on the day of delivery”, which leads me to my first topic: materials. We tend to avoid most metals because seawater is highly corrosive. So we use plastics, glass, carbon, fiber-reinforced plastics and high-grade stainless steel. Whenever the lack of alternatives justifies the extremely high costs, we use titanium, a high-tech metal stronger than steel while being only half as heavy and absolutely corrosion-resistant.
Speaking of titanic strength brings me to the next significant challenge in deep-sea technology: withstanding pressure. Pressure compresses gas (it also compresses liquids and solids but to a much lower extent). So if there is air in a housing when you bring it under water, it will either fill with water or implode. Therefore, we fill the housings of electronics, sensors and some battery types with oil so that there is no air left inside to be compressed. There are, however, components that have hollow spaces which cannot be filled with oil, such as cameras or optical sensors, because oil has different optical properties than the air these devices are made to function in. In this case, we use titanium to build pressure-proof housings that keep the inside dry and at atmospheric pressure.
Another major challenge is under-water communication. Seawater does neither transport GPS signals, nor radio communication, mobile networks or WiFi. The only way to communicate with a submerged device through water over a long distance is through ultrasound communication. The data rate and reliability of such communication is, however, just about sufficient to transmit “Time to come back up!” and receive the answer “Ok, I’m on my way!”. So either you accept that you have little to no communication with your sensor, your experiment setup, or your robot, or you connect it physically to your computer via a cable. For this purpose, RV SONNE is equipped with a whole family of cranes, winch systems and cables, each of several thousand meters in length, some of which can transfer electrical energy at more than a thousand volts. Live HD video transmission from the seafloor and remote operation of deep sea robots such as the ROV Kiel 6000 would not be possible without this high-tech cable infrastructure. Through the ROV, even devices without a cable of their own can be remotely operated, for example through magnet switches or short-distance optical barcode-communication modules.
Not only rudimentary communication is supported by ultrasound, but also positioning data. The RV SONNE has ultrasound transponders that can locate their counterparts that are attached to the devices we bring to the sea floor. We add this relative position to the ship to the GPS position of the ship so that we can mark the absolute coordinates of our device on a map to find it again later.
Scientific interests of MPI/AWI: Our MPI/AWI joint research group studies the abundance and consumption of oxygen in deep-sea sediment. As on land, many deep-sea organisms depend on oxygen. As opposed to life on land or in shallow water, where plants and some bacteria can produce oxygen through photosynthesis using sunlight, the deep sea is dark. So life depends on the oxygen dissolved in the surface water and brought down to the seafloor by the global ocean conveyor belt. This oxygen then again diffuses slowly into the sediment, where it is consumed biogeochemically mediated by living organisms.
With our research, we measure high-resolution vertical profiles of oxygen concentration in the upper sediment layer (< 30 cm) as well as high-precision oxygen diffusion and consumption rates on the seabed.
Sensitive sensors: Now that you are equipped with a basic understanding of deep-sea technology as well as our research interest, I can explain our devices to you.
High precision and high resolution of our measurements are specifically crucial to our research. But by pushing sensors into fluffy sediment, we disturb the natural layering, we want to collect our data in. So we have to keep the physical impact on the sediment as gentle as possible. Therefore, we use different types of glass needles with a sensor tip of 20, respectively 240 micrometer in diameter, which is about as thin as a human hair. These needles are custom-made by technicians in our labs in Bremen.
So-called fiber-optical deep profilers immerse 30 centimeters into the sediment. The glass needles are splinted with a rigid carbon tubing ending in a medical cannula protecting the tip. This tip is coated with a delicate layer of paint that turns fluorescent in the presence of oxygen. For the measurement, a ray of light is sent through the glass fibre and reflected at the tip. The intensity of the light at the specific fluorescence wavelength that travels back through the glass fiber is measured to calculate the oxygen concentration around the tip.
Our electrochemical mirco-profilers, on the other hand, pierce only the upper 15 centimeters of the sediment. Their glass needles are hollow and support two thin filaments maintaining a low electrical voltage. When in contact with oxygen, a very low electrical current flows between the two filament electrodes. The oxygen concentration can then be calculated from the measured electrical current.
Both types of profilers have several elements in common, as shown in figure 1: (1) a rigid frame of fiber-reinforced plastics, (2) a pressure-proof titanium housing containing the main controller and the sensor electronics where the optical, respectively electrochemical signal is received, interpreted and logged, (3) a custom-made oil-filled battery for power supply, (4) an optical communication module for close-up communication with the ROV, (5) custom-made oil-filled motors and gear mechanics for driving the needles vertically, and (6) a knotted monkey fist handle for lifting and positioning by the ROV.
My daily work: My responsibility as a mechanical engineer in the team is to make sure these devices work mechanically. Programming, sensor calibration and data interpretation is being done by our boss, Frank, in the lab. My main workspace is the ship’s hangar, the workshop hall on deck, where I have all my tools and spare parts to repair whatever breaks, and the charging stations for the batteries. And here is what I find most challenging, but fascinating about my job: 1) It is indeed an educational trip for creative improvisation. I can only work with whatever we brought onto this ship, we cannot buy spare parts or material. 2) I always have to take great care not to damage our delicate sensors. 3) I still feel excited every time I see my devices dive deep down into the ocean and yet even more relieved when they come back up unscathed, carrying home a bunch of precious data …
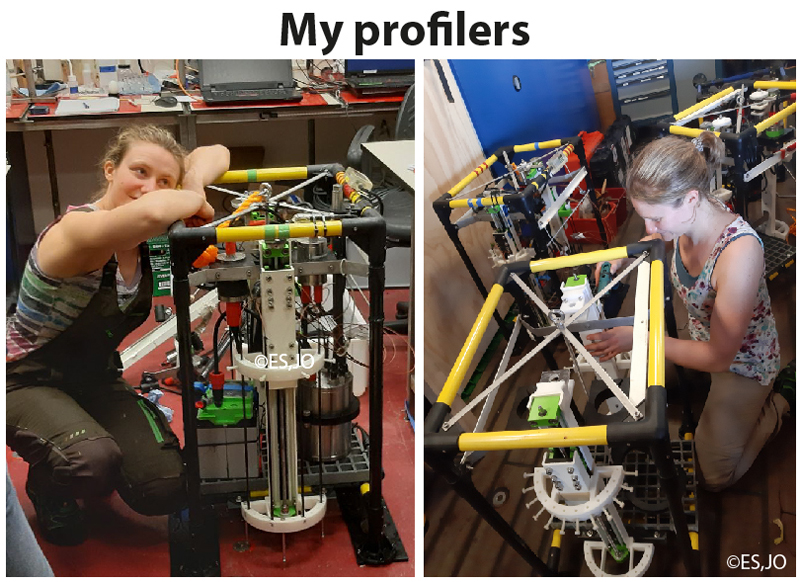
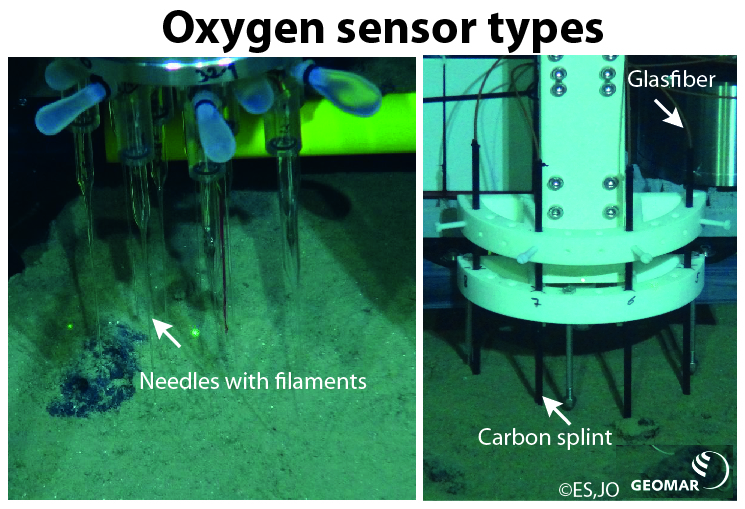
von Elena Schiller
Grundlagen der Tiefseeforschungs-Technologie: Das Erste, was ich in einer Tiefseetechnik-Werkstatt gelernt habe, ist “Rostfreier Stahl ist rostfrei am Tag der Anlieferung”, was mich direkt zum ersten Thema bringt: Materialien. Wir vermeiden die meisten Metalle, da Seewasser hoch korrosiv ist. Am häufigsten verwenden wir Plastik, Glas, Karbon, faserverstärkte Kunststoffe und hochwertigen Edelstahl. Wann immer der Mangel an Alternativen die enorm hohen Kosten rechtfertigt, verwenden wir Titan, ein high-tech-Metall, fester als Stahl, dabei aber nur halb so schwer und absolut korrosionsbeständig.
Titanische Stärke bringt mich schon zur nächsten großen Herausforderung in der Tiefseetechnik: dem Druck standzuhalten. Druck komprimiert Gase (Er komprimiert auch Flüssigkeiten und Feststoffe, aber in viel geringerem Maße). Wenn also Luft in einem Gehäuse ist, wird sich dieses Gehäuse beim Abtauchen entweder mit Wasser füllen oder implodieren. Daher füllen wir die Gehäuse einiger Elektronik-Elemente, Sensoren und einiger Batterietypen mit Öl, sodass keine Luft mehr darin ist, die komprimiert werden könnte. Es gibt jedoch Einzelteile, die Hohlräume besitzen, die nicht mit Öl gefüllt werden können. Dazu gehören zum Beispiel Kameras oder optische Sensoren, da Öl andere optische Eigenschaften besitzt als Luft, für die sie gebaut sind. In diesen Fällen bauen wir druckfeste Gehäuse aus Titan, die ihr Inneres trocken und auf einem Bar atmosphärischem Druck halten.
Eine weitere Herausforderung ist die Unterwasser-Kommunikation. Meerwasser leitet keine GPS-Signale, keinen Funk, keine Telefon-Strahlung und kein WLAN.
Der einzige Weg, durch das Wasser über lange Distanz mit einem getauchten Gerät zu kommunizieren ist über Ultraschall. Die Datenrate und Zuverlässigkeit solcher Kommunikation ist aber gerade so eben ausreichend, um zu senden “Zeit, wieder hoch zu kommen!” und die Antwort zu erhalten “Alles klar, bin unterwegs!”. Also entweder akzeptiert man, dass man sehr wenig bis gar keine Kommunikation zu seinem Sensor, Experiment-Setup, oder Roboter aufbauen kann, oder man verbindet sich physisch mit ihm über ein Kabel. Zu diesem Zweck ist FS SONNE ausgestattet mit einer ganzen Familie von Kränen, Windensystemen und Kabeln, je mehrere Kilometer lang, von denen einige elektrische Energie mit einer Spannung von über 1000 Volt übertragen können. Live HD Videoübertragung vom Meeresgrund und Fernsteuerung von Tiefsee-Robotern wie das ROV Kiel 6000 wären ohne diese high-tech Kabel-Infrastruktur nicht möglich. Mit Hilfe des ROVs können auch Geräte, die selbst nicht an einem Kabel hängen, ferngesteuert werden, etwa durch Magnetschalter oder Module zur optischen Kommunikation über Barcodes.
Ultraschall wird jedoch nicht nur zu rudimentärer Kommunikation, sondern auch zur Positionsbestimmung getauchter Geräte verwendet. FS SONNE trägt Ultraschall-Transponder, die ihre Gegenstücke orten können, die wir an unseren Tiefsee-Geräten befestigen. Wir summieren diese relative Position zum Schiff auf die absoluten GPS-Koordinaten des Schiffes, um die absolute Position unserer Geräte zu kennen. Auf diese Weise können wir sie später wiederfinden.
Wissenschaftliche Fragestellung des MPI/AWI: Unsere MPI/AWI Brückengruppe erforscht unter anderem die Verfügbarkeit und den Verbrauch von Sauerstoff in den obersten Sedimentschichten. So wie an Land nutzen auch in der Tiefsee viele Organismen Sauerstoff zum Überleben. Im Gegensatz zum Leben an Land oder in flacherem Gewässer, wo Pflanzen und einige Bakterien Sauerstoff herstellen können, indem sie mit Hilfe von Sonnenlicht Photosynthese betreiben, ist es in der Tiefsee dunkel. Das Leben dort ist also angewiesen auf den Sauerstoff, den es aus dem Wasser beziehen kann. Dieser Sauerstoff wiederum diffundiert langsam in das Sediment, wo er einerseits biochemisch von Organismen und andererseits durch geochemische Prozesse abgebaut wird. Für unserer Forschung messen wir hochauflösende vertikale Profile der Sauerstoffkonzentration in den obersten Sedimentschichten (< 30 cm) sowie hochpräzise Diffusions- und Verbrauchsraten von Sauerstoff.
Empfindliche Sensoren: Jetzt, da Ihr mit einem grundlegenden Verständnis von Tiefsee-Technik und auch von unserem Forschungsgebiet ausgestattet seid, kann ich Euch unsere Geräte erklären.
Hohe Präzision und Auflösung ist speziell für unsere Forschung von großer Bedeutung. Indem wir Sensoren in lockeres Sediment drücken, zerstören wir aber die natürliche Schichtung, die wir ja gerade erkennen möchten in unseren Daten. Wir müssen also die physikalische Beeinflussung des Sediments so gering wie möglich halten. Daher verwenden wir verschiedene Arten von Glasnadeln mit je einer Sensor-Spitze von 20 bzw. 240 micrometer Durchmesser (das ist etwa so fein wie ein menschliches Haar). Diese Nadeln werden handgemacht von kompetenten technischen Assistent*innen am MPI in Bremen.
Sogenannte Faser-optische tiefe Profiler stechen 30 cm tief ins Sediment. Die Glasnadeln sind geschient in einem stabilen Röhrchen aus Karbon, das in einer medizinischen Kanüle endet, die die Spitze der Glasnadel schützt. Diese Spitze ist lackiert mit einer empfindlichen Schicht Farbe, die bei Kontakt mit Sauerstoff fluoreszierend wird. Bei der Messung wird ein Lichtstrahl durch die Glasfaser in Richtung Sediment geschickt und an der lackierten Spitze reflektiert. Die Intensität des zurückgestrahlten Lichts in der Wellenlänge der Fluoreszenz durch Sauerstoff wird gemessen, um daraus die Sauerstoffkonzentration um die Spitze herum zu berechnen.
Unsere elektrochemischen Micro-Profiler andererseits tauchen nur 15 cm tief ins Sediment ein. Ihre Glasnadeln sind hohl und ummanteln je zwei feine Drähte, an denen eine geringe elektrische Spannung anliegt. Bei Kontakt mit Sauerstoff fließt ein geringer elektrischer Strom zwischen den Drähten. Die Sauerstoffkonzentration um die Spitze kann aus der Höhe dieses elektrischen Stroms berechnet werden. Beide Profiler-Typen haben einige Elemente gemein: (1) Ein stabiler Rahmen aus faserverstärktem Kunststoff, (2) ein Druckgehäuse aus Titan, das den Main Controller beherbergt sowie die Sensorelektronik, an der die optischen bzw. elektrochemischen Signale aufgenommen, interpretiert und geloggt werden, (3) eine spezialangefertigte, durch Öl-Füllung druckkompensierte Batterie zur Stromversorgung (4) ein optisches Modul für Kommunikation mit dem ROV auf kurze Distanz, (5) spezialangefertigte, durch Öl-Füllung druckkompensierte Motoren mit Mechanik zum Herunterfahren der Sensoren und (6) eine geknotete Affenfaust als Griff für das ROV zum Anheben und Absetzen.
Mein Arbeitsalltag: Als Mechanik-Ingenieurin ist es meine Aufgabe, dafür zu sorgen, dass unsere Geräte mechanisch funktionieren. Das Programmieren, die Kalibrierung der Sensoren und die Interpretation der Daten übernimmt mein Chef, Frank, im Labor. Mein hauptsächlicher Arbeitsplatz hier auf dem Schiff ist der Hangar, also die Werkstatt-Halle an Deck. Dort habe ich neben den Ladestationen für die Batterien Werkzeug und Ersatzteile, um zu reparieren, was so kaputt geht. Zuletzt noch ein paar Worte, was ich an diesem Job besonders anspruchsvoll, aber auch faszinierend finde: 1) Eine Schiffsausfahrt ist ein Lerncamp zur kreativen Improvisation. Ich kann nur mit dem arbeiten, was wir mit an Bord genommen haben. Ich kann kein Material, Werkzeug oder Ersatzteile kaufen. 2) Ich muss sehr sorgsam arbeiten, um die empfindlichen Sensoren nicht zu beschädigen. 3) Es ist immer wieder aufregend, meine Geräte ins tiefe Wasser zu lassen und immer wieder ebenso erleichternd, wenn sie heil und mit wertvollen Daten im Gepäck wieder nach Hause kommen …