(English below)
Manganknollen – Entstehung und wirtschaftliche Relevanz
von Jessica Volz, Sophie Paul und Julia M. Otte
Während der ersten globalen Forschungsexpedition der HMS Challenger in den 1870er Jahren wurde das große Ressourcenpotential der Tiefsee deutlich. An Bord der HMS Challenger wurden zahlreiche unbekannte marine Organismen sowie mineralische Konkretionen vom pazifischen und atlantischen Meeresboden, die wie schwarzer kleiner Blumenkohl aussehen, gewonnen – dabei handelte es sich um Manganknollen (Abbildung 1, oben). Im Zuge nachfolgender Forschungsexpeditionen in den Weltmeeren wurde eine Vielzahl von Mineralvorkommen in der Tiefsee entdeckt, darunter Mangankollen-Felder, massive Sulfide und kobaltreiche Krusten.
Das größte bekannte Vorkommen von Manganknollen mit signifikanten Mengen an Kupfer (Cu), Kobalt (Co) und Nickel (Ni) befindet sich in der Clarion-Clipperton-Fracture-Zone (CCZ) im nordöstlichen Pazifik zwischen Hawaii und Mexiko – einer Fläche etwa der Größe Europas (Abbildung 3). Da viele Metalle, die für die heutigen Hightech-Entwicklungen benötigt werden, in Mangankollen enthalten sind, wächst das wirtschaftliche Interesse an den Vorkommen in der Tiefsee. Die gesellschaftliche Nachfrage nach z.B. Kupfer, Lithium und Seltenen Erden steigt durch den zunehmenden Einsatz von Handys, Laptops und Flachbildfernsehern, sowie durch das Wachstum des Sektors der erneuerbaren Energien: Photovoltaikzellen, Magnete in Windkraftanlagen und Batterien für Elektroautos sind auf nicht-erneuerbare Ressourcen angewiesen. Der kontinuierliche Wirtschaftswachstum und die Erschöpfung terrestrischer Erzvorkommen treiben die Erkundung alternativer Rohstoffvorkommen voran, da das Recyclingpotenzial kritischer Materialien bislang noch begrenzt ist.
Seit der Entdeckung der Manganknollen-Felder der CCZ haben zahlreiche Studien die Herkunft, Bildung und das Ressourcenpotential der Knollen untersucht. Da sich der Meeresboden in der CCZ in mehr als 4000 m Wassertiefe befindet, werden hier nur geringe Mengen an Sedimenten von weniger als einem Zentimeter über tausend Jahre abgelagert. Diese sehr geringe Ablagerungsrate der Sedimenten erlaubt das Vorkommen und Wachstum von Manganknollen auf dem Meeresboden. Die Bildung der Knollen erfordert einen Wachstumskern (Nucleus), etwa Fragmente von Vulkangestein oder biogenes Material wie Haizähne oder Stücke von Muschelschalen. Die Hauptbestandteile der Manganknollen – Eisen und Mangan – bilden Oxid-Schichten in sauerstoff-reichem (oxischem) Meerwasser am Meeresboden um den Nucleus herum.
Während dieses Entstehungsprozesses werden verschiedene Metalle aus dem Meerwasser an die negativ geladenen Oberflächen von Manganoxiden (MnO2) und positiv geladenen Oberflächen von Eisen(oxyhydr)oxiden (FeOOH) adsorbiert, was zur Metallanreicherung in den Knollen führt (Abbildung 2). Dieser Wachstumspfad bei dem Metall aus der Wassersäule absorbiert wird, wird als “hydrogenetisches Wachstum” bezeichnet, bei dem die Knollen über eine Million Jahre hinweg etwa 2 Millimeter wachsen.
Die meisten Manganknollen der CCZ zeigen Schichten unterschiedlicher Herkunft und Wachstumsraten. Die Knollen werden nicht nur mit Metallen aus dem Meerwasser gespeist, sondern absorbieren auch Metalle, die beim mikrobiellen Abbau organischer Substanz in den Sedimenten unterhalb der Knollen freigesetzt werden. Da die Oberflächensedimente des CCZ in der Regel reich an Sauerstoff sind, wird die Anreicherung von Metallen durch den Abbau organischer Substanz als “oxisches diagenetisches Wachstum” bezeichnet. Unter bestimmten Bedingungen im Ozean in der Vergangenheit wurden die Knollen mit Metallen aus tieferen Sedimenten gespeist, die durch das zwischenliegende Porenwasser angeliefert wurden. Während dieses so genannten “suboxischen diagenetischen Wachstums” wachsen die Knollen schneller mit bis zu 250 Millimetern in einer Million Jahre. Für das suboxische diagenetische Wachstum der Knollen muss Sauerstoff weniger tief in die Sedimente eingedrungen sein, als es heute in der CCZ beobachtet wird.
Zusätzlich zu den abiotischen (chemischen) Knollenbildungspfaden zeigen neue Studien, dass Manganknollen einen einzigartigen Lebensraum für prokaryotische Gemeinschaften bieten. Es ist bekannt, dass Knollen-spezifische Gemeinschaften von sowohl Mangan-/Eisen-Reduzierern also auch von –Oxidierern dominiert werden und für den Mangan- und Eisenzyklus der Knollen eine wichtige Rolle spielen könnten (Abbildung 3). Dieser biologisch gesteuerte Metallkreislauf ist bisher noch nicht gut verstanden und könnte für die Bildung der Manganknollen relevant sein, da die Bakterien aktiv Mangan- und Eisen(oxyhydr)oxide produzieren.
Im Rahmen von MiningImpact wollen wir einen Beitrag zum Verständnis der Bildung und Verteilung von Manganknollen leisten, indem die Sediment-Geochemie und mikrobielle Gemeinschaften in Knollen und Sedimenten untersucht werden. Die Rolle der Manganknollen als natürlichen Lebensraum für Tiefseeökosysteme stellt eine weitere wichtige Grundlagenstudie für die Bewertung möglicher zukünftiger Auswirkungen des Bergbaus dar.
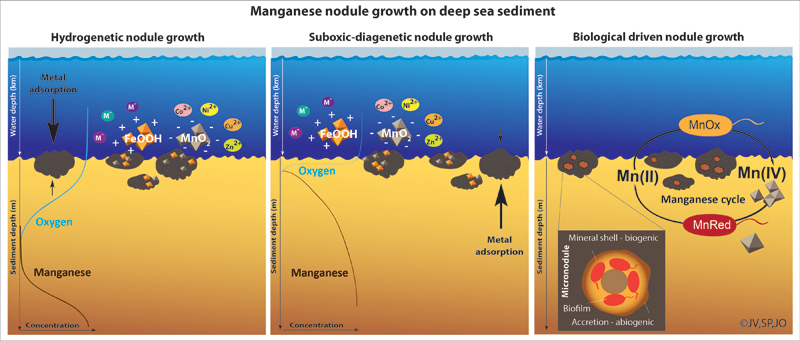
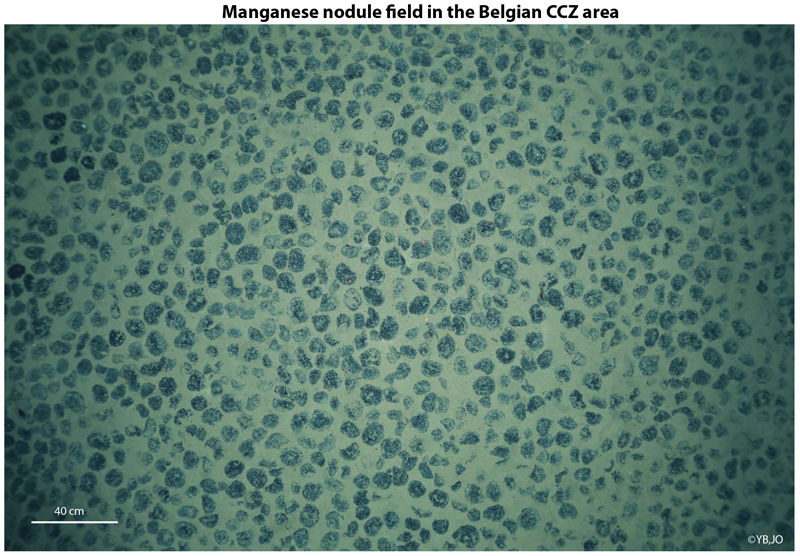
Polymetallic Nodules – Formation and economic value of manganese nodules
by Jessica Volz, Sophie Paul and Julia M. Otte
The great resource potential of the deep sea was discovered during the first global marine research voyage conducted by the HMS Challenger in the 1870s. During the Challenger expedition, numerous unknown marine species of fauna were collected as well as potato-shaped mineral concretions in the Atlantic and Pacific Ocean: polymetallic nodules (Figure 1).
In the course of continuing marine research throughout the world ocean, a variety of deep-sea mineral deposits were discovered, including fields of polymetallic nodules, seafloor massive sulfides, and cobalt-rich crusts. The most extensive deposit of polymetallic nodules with economically viable amounts of copper (Cu), cobalt (Co), and nickel (Ni) is known in the Clarion-Clipperton Fracture Zone (CCZ) in the northeast Pacific Ocean between Hawaii and Mexico – an area approximately of the size of Europe.
As many metals needed for today’s high- and green-tech developments are contained in polymetallic nodules, the economic interest in polymetallic nodules is thriving. Societal demand for e.g. copper, lithium, and rare earth elements is increasing due to the extensive use of cell phones, laptops, and flat screen TVs as well as the growth of the renewable energy sector: photovoltaic cells, magnets in wind turbines, and electric car batteries depend on non-renewable resources. Continuous economic growth and the depletion of high-grade terrestrial ore deposits drive the exploration of alternative ore deposits as the recycling potential of critical materials is still limited.
Since the discovery of deep-sea polymetallic nodule fields in the CCZ, numerous studies have focused on understanding their origin and assessing their resource potential. As the seafloor in the area of the CCZ is located at more than 4000 m water depth, only low amounts of sediment are deposited on the seafloor at a rate of less than one centimeter over a thousand years. This very low accumulation rate of sediments on the seafloor facilitates the growth of polymetallic nodules.
The formation of polymetallic nodules requires a growth core (nucleus), which can be fragments of volcanic rocks or biogenic material, such as shark teeth or pieces of clam shells. The main constituents of polymetallic nodules – iron and manganese – form layers of oxides around the nucleus in oxygen-rich (oxic) seawater at the seafloor. During this formation process, various metals are adsorbed from the seawater onto the negatively charged surfaces of manganese oxides (MnO2) and positively charged surfaces of iron oxyhydroxides (FeOOH), leading to the nodule’s metal enrichment (Figure 2).
This growth pathway of metal adsorption from the water column is referred to as “hydrogenetic growth” during which the nodules grow about two millimeter over a million years. Most polymetallic nodules found in the CCZ show growth layers of different origin and growth rates. The nodules are not only fed with metals from the seawater but also adsorb metals, which are released in the sediments beneath the nodules during the microbial degradation of organic matter. As the surface sediments of the CCZ are usually rich in oxygen, the accretion of metals through the degradation of organic matter is called “oxic diagenetic growth”.
Under specific environmental conditions in the ocean in the past, the nodules were also supplied with metals delivered from deeper sediments by the interstitial pore water. During this so called “suboxic diagenetic growth”, the nodules grow much faster with up to 250 millimeter over a million years. In order to facilitate the suboxic diagenetic nodule growth, oxygen must have penetrated less deep into the sediments than it is observed today in the CCZ (Figure 2).
In addition to the abiotic (chemical) nodule formation pathways, recent studies revealed that manganese nodules provide a unique habitat for prokaryotic communities. It is known that the nodule-specific community is dominated by members of manganese- and iron-cycling microorganisms and might play an important role for the cycling of manganese and iron on and inside of the nodules (Figure 3). This biologically driven metal cycle is not yet fully understood but could be relevant for the polymetallic nodule formation as the bacteria actively produce manganese and iron oxyhydroxides.
As part of the MiningImpact project, we aim to improve our understanding of the formation and distribution of polymetallic nodules by studying the sediment geochemistry as well as the involved microbial community on nodules and in the underlying sediments. The role of manganese nodules as habitats for deep-sea ecosystems represents a further crucial baseline study for the evaluation of potential future mining impacts.